Coding Principles of Dopaminergic and Cholinergic Systems in the Brain
1) The Dopamine Release
Dopamine neurons represent less than 0.01% of neurons in the brain, but are essential for controlling several key brain functions. While studies over the past decades have generated a wealth of knowledge on the importance of dopamine, fundamental knowledge gaps exist regarding its signaling. Unlike fast point-to-point communication, most dopamine terminals form “open” synapses without a clear postsynaptic side. It has long been proposed that dopamine release happens randomly on the axonal membrane without strict spatiotemporal precision. We have challenged this doctrine by finding two key features of dopamine release: 1) Most dopamine release only happens at some “hot spots” that contains active zone-like structures along the axon. 2) Dopamine release exhibit extremely high release probability. These findings suggest that dopamine signaling actually contains an elegant signaling architecture with high spatial and temporal precision. Our research in this direction focues on the physical and molecular basis of dopamine release, subcellular organization of dopamine transmission and network regulation of dopamine signaling.
Reference: Liu, et al. Cell 2018, Liu, et al. Nature Reviews Neuroscience 2021, Liu, et al. Current Opinion in Neurobiology 2019
Super-resolution imaging of active zones in dopamine axons. Rotating 3D view of bassoon (green, active zone marker) and TH (magenta, dopamine axon marker) from a 3D-SIM experiment in a dorsal striatal slice (10x10x2 μm3) showing volume-rendered raw images (1-10s), surface-rendered bassoon clusters and dopamine axons (10-17s) and bassoon clusters within dopamine axon.
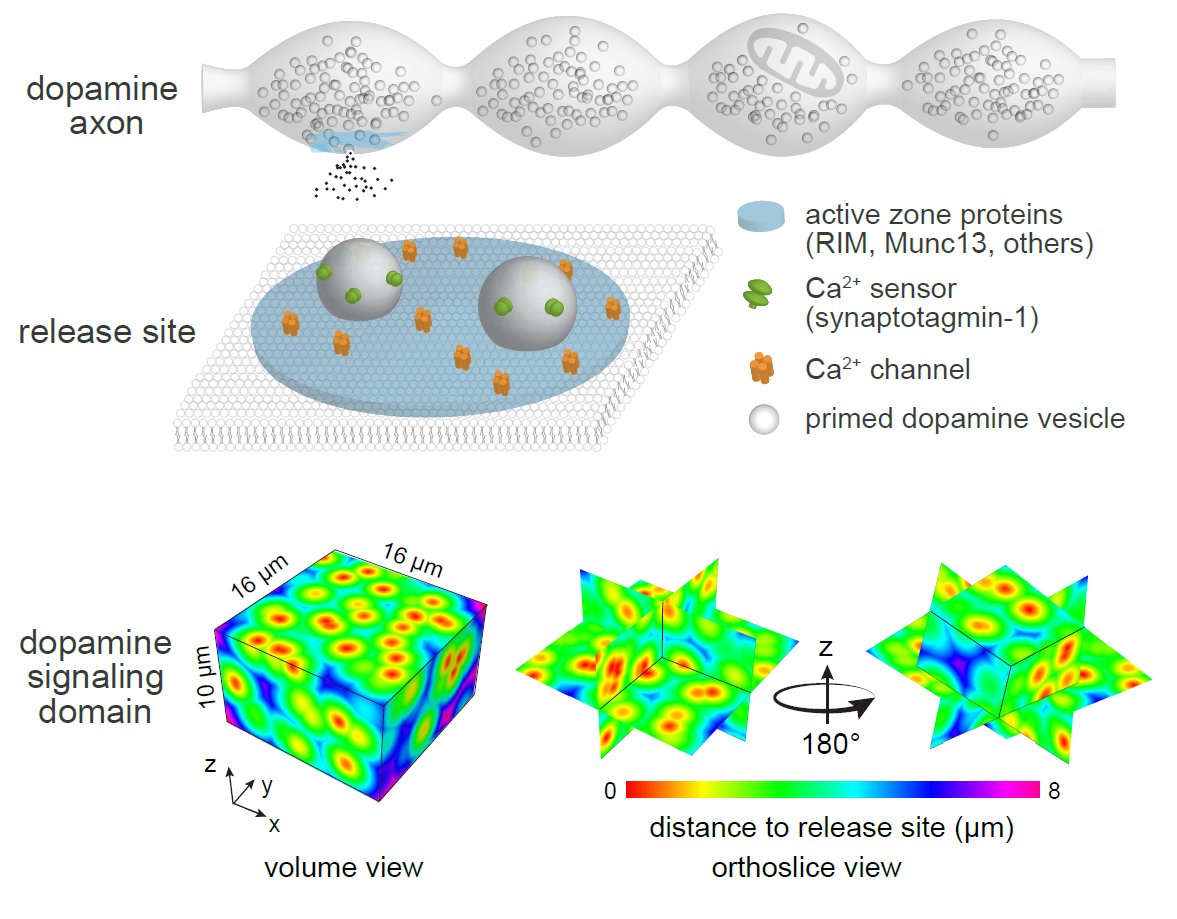
Dopamine release sites and release mode. Top, Cellular and molecular organization of dopamine release sites. Only ~25% of dopamine varicosities contain functional release sites composed of active-zone proteins. Bottom, Distribution of release sites and the impact area of individual dopamine release events in the striatum. The sparsity of active zone-containing varicosities, the long-lasting depression of individual sites after a release even and the rapid dilution of dopamine into the extracellular space suggest that, at any given time, only a small fraction of the space reaches high-enough dopamine levels for efficient receptor activation.
2) The Interaction Between Dopamine and Acetylcholine
Information flow in neurons proceeds by integrating inputs in dendrites, generating action potentials in the soma and releasing neurotransmitters in the axon. We found that the activity of striatal cholinergic interneurons induces action potential firing in distal dopamine axons and broadcasts dopamine release. This mechanism bypasses the law of dynamic polarization of dendrite-soma-axon information flow, serves as a physiological regulation for striatal computation and contributes to behavior. Meanwhile, released dopamine strongly inhibits the activity of cholinergic neurons with high spatiotemporal precision. The purpose of this study is to understand the prevalence, physical basis, functional significance, and therapeutic potentials of these reciprocal interactions.
Reference: Liu, et al. Science 2022
Spontaneous ACh-induced dopamine release in striatal slices. Example imaging and analyses of dopamine release (using DA sensor) in striatal slices (10 s, normal playback speed). A raw movie is shown on the left, the corresponding ΔF/F0 is shown in the middle, and detected dopamine release events are depicted on the right.

Acetylcholine induces ectopic firing in dopamine axons. Left, Schematic and example two-photon image of direct recording from dopamine axons. Synaptophysin-tdTomato was expressed by using mouse genetics in dopamine axons. The recorded axon was filled with Atto488 (green) through the recording pipette and puff pipette containing carbachol (acetylcholine receptor agonist) and Atto 488. Middle, Example responses of dopamine axon to current injections through the whole-cell pipette. Right, responses of the recorded dopamine axon to 10 consecutive carbachol puffs. Axonal action potentials were evoked using this stimulation.
3) The Principles of Motor Control in the Striatum
The basic organization of the striatal circuits involves two fundamental pathways: the direct pathway is composed of dopamine D1 receptor-expressing medium spiny neurons (D1-MSNs), and the indirect pathway is based on D2 receptor-expressing MSNs (D2-MSNs). Movement is thought to result from the dynamic balance of the two pathways that are finely tuned by dopamine and acetylcholine. We have found that striatal dopamine and acetylcholine levels are highly correlated with movement direction, with an increase when the animal is moving forward or turning contralaterally and a decrease if the animal is moving towards the opposite directions. This research aims to reveal how the pattern evolves, how dopamine and acetylcholine regulate the two striatal pathways, and how these systems coordinate to give rise to spontaneous moving dynamics.
Reference: Liu, et al. Science 2022
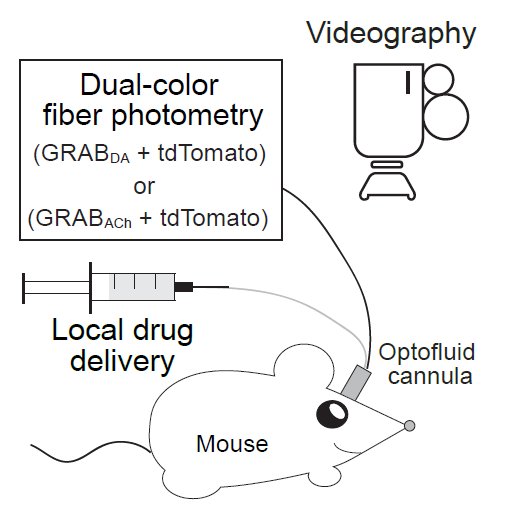

Dopamine and acetylcholine dynamics correlate with movement direction. Left, Strategy for simultaneous measurements of dopamine and acetylcholine dynamics and behavior in freely moving mice. Middle, An example video clip demonstrating animal movement and concurrent dopamine levels in the dorsal striatum (color-coded in the cube). Right, Average dopamine and acetylcholine levels registered to their concurrent velocities in polar coordinates.
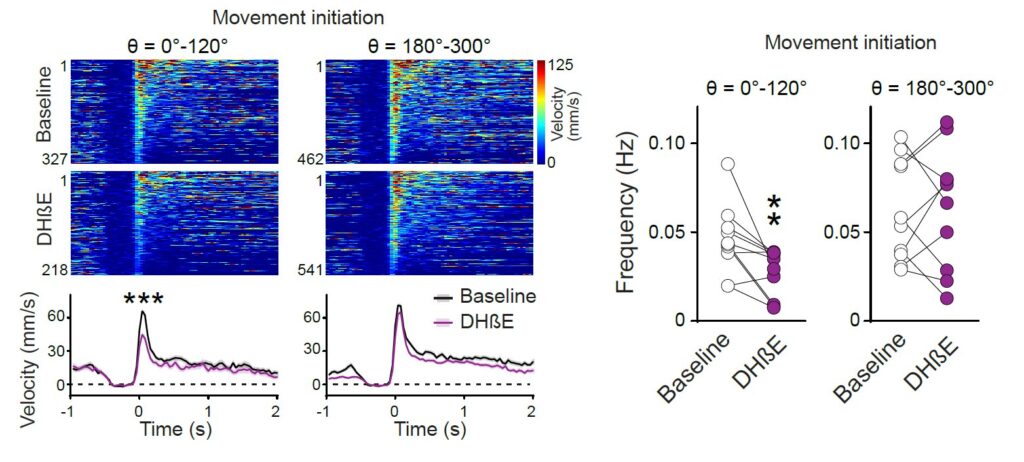
Local inhibition of nAChRs in the striatum alters moving patterns. Left, Individual (heatmaps) and average time course of velocity amplitude during movement initiations before and after local infusion of nAChR blocker DHβE into the right dorsal striatum at the recording site. This process caused a significant reduction in the velocity amplitude and frequency of movement initiations in specific directions.
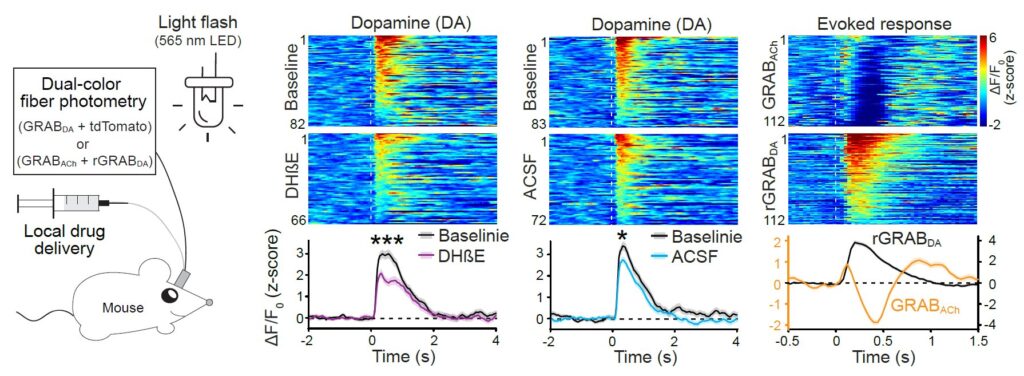
Acetylcholine enhances dopamine release in vivo. Left, Schematic for measurements of dopamine release induced by 200-ms light flashes in freely moving mice. Right, Individual (heatmap) and average dopamine and acetylcholine release aligned to the light flash (dashed line). Acetylcholine release precedes dopamine release and is immediately suppressed by dopamine once dopamine is released. nAChR blocker DHβE reduces the light-evoked dopamine release.